I’ve argued that obesity, diabetes and heart disease are the human expression of mammalian torpor, an altered metabolic state characterized by high levels of desaturase enzymes and high circulating branched chain amino acids (BCAAs): leucine, isoleucine and valine. A torpid mammal prefers to store fat rather than burn it.
Two 2023 papers add significant evidence that human metabolic syndrome ”looks like” mammalian torpor. Let’s start with BCAAs.
BCAAs are Elevated in Torpor and Obesity
The first paper looks at the metabolome of the thirteen lined ground squirrel, a North American hibernator. The squirrels were sampled in Spetember, during the active season, again in October as they approached torpor then again when fully torpid (TOR). Another time point is ”Interbout Arousal(IBA)” – brief periods when the animals raise their body temperature between torpor bouts lasting up to two weeks. The last time period is March – soon after torpor when it is still very cold where these squirrels live.
Red means high levels and blue is low levels. You can clearly see that leucine and isoleucine hit their peak levels during full blown torpor. I’ve added helpful red arrows to emphasize the trend.
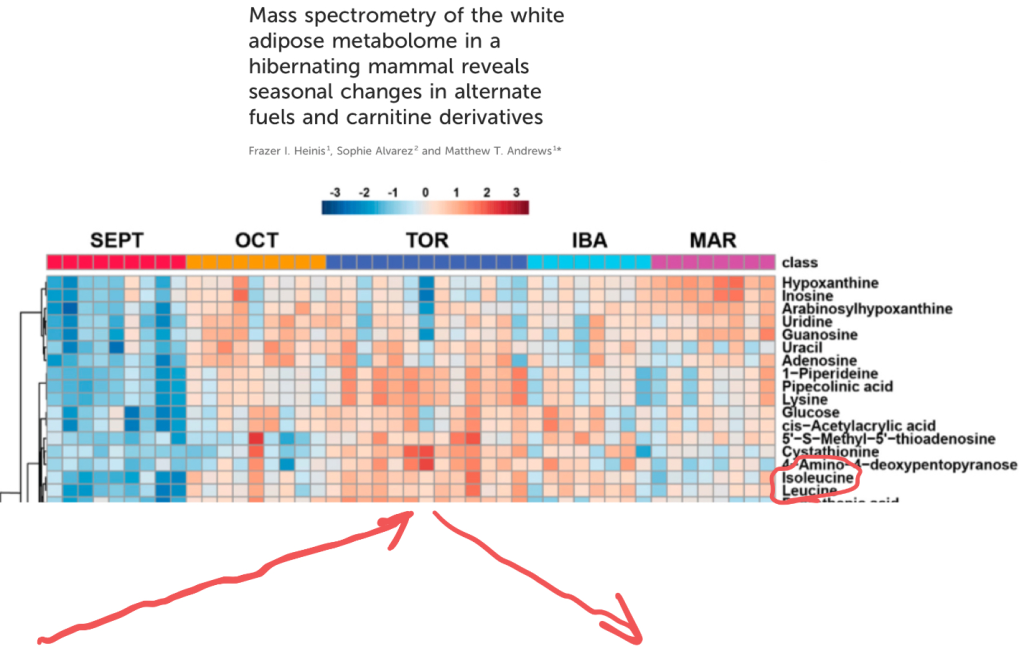
This mirrors the state in human obesity, reported all the way back in 1969.
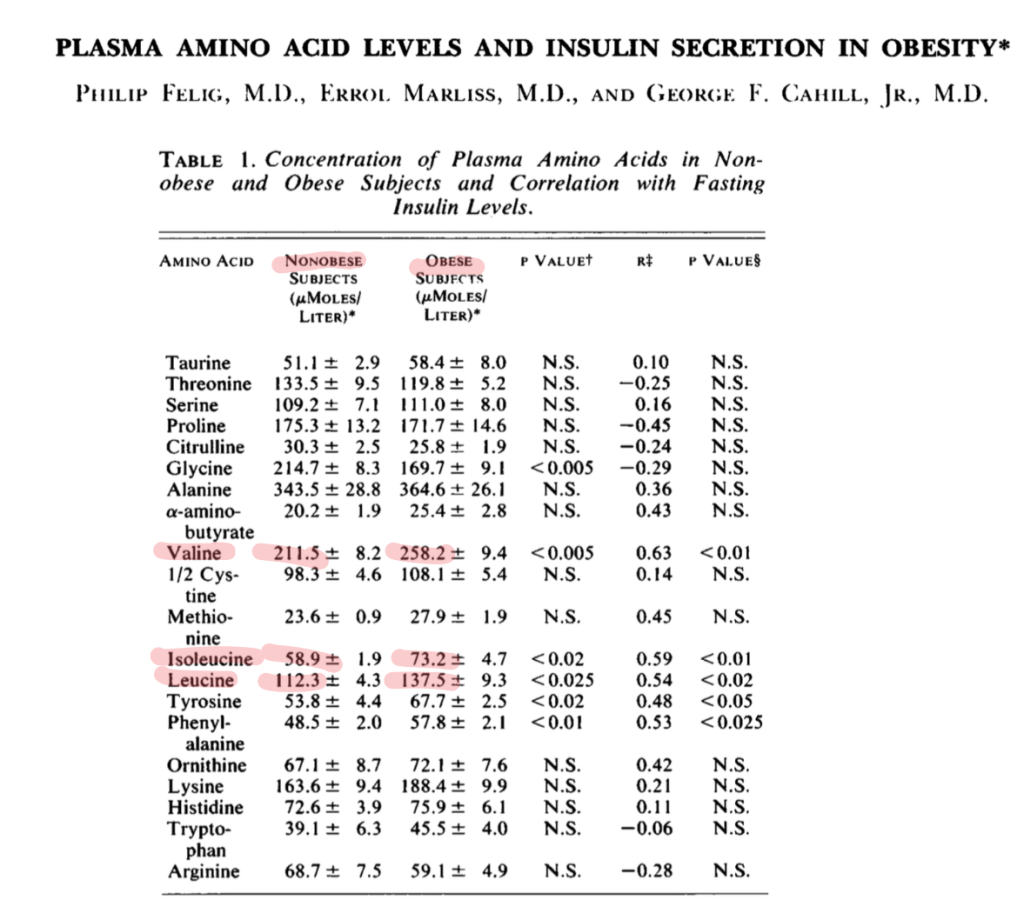
Hibernating animals have remarkable abilities to conserve muscle. One of the reasons for this is presumably the fact that they downregulate the enzyme branched chain ketoacid dehydrogenase (BCKDH). BCKDH catalyzes the limiting step in breaking down BCAAs.
This paper shows the big decrease in BCKDH levels in arctic ground squirrels in torpor versus post-reporduction (PR) – the active part of their season.
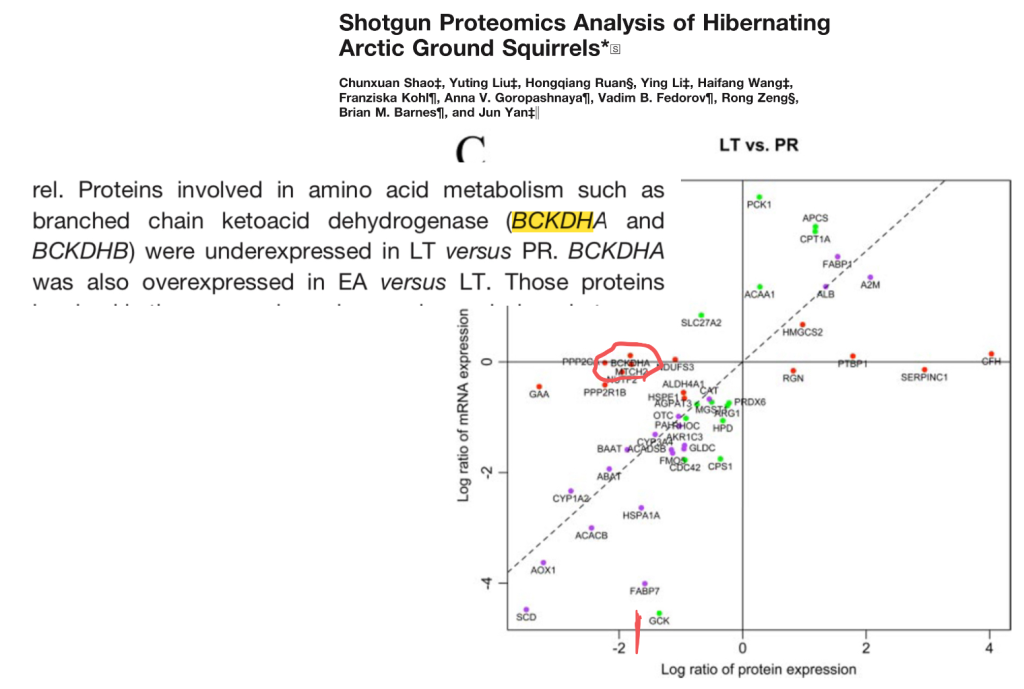
This is also reflected in human obesity. This study looked at the levels of expression of BCKDH in lean vs. obese Pima Indians in men and women.
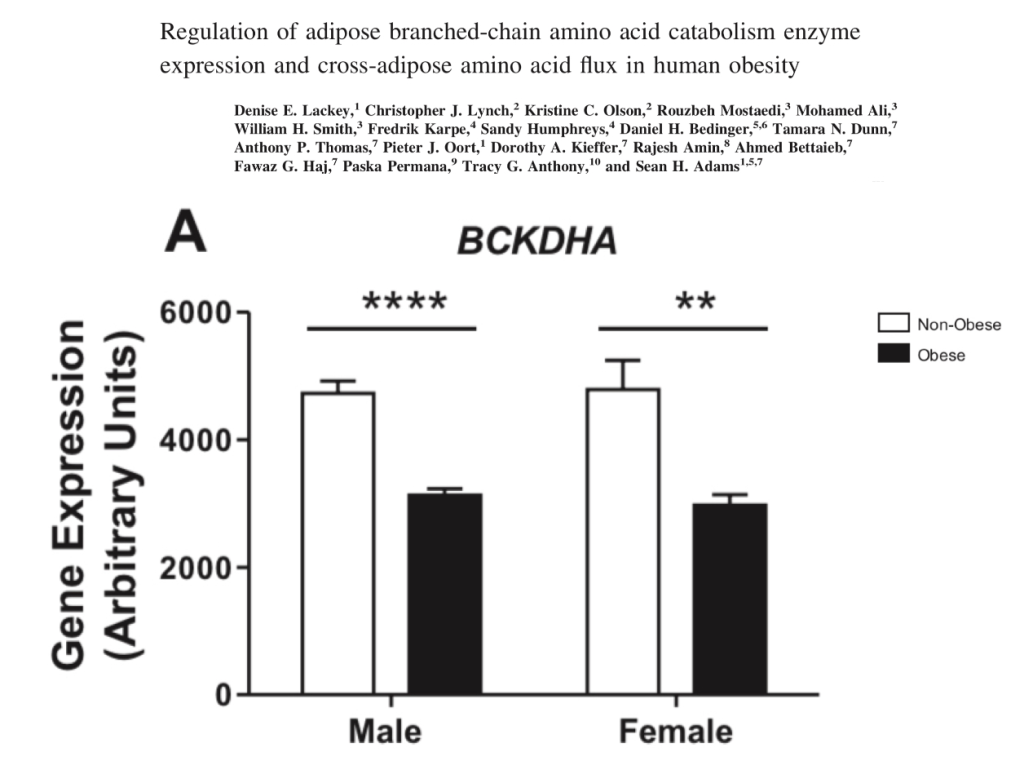
We can say that torpid animals and obese humans share the characteristics of increased circulating BCAAs and decreased BCAA catabolism.
UPDATE: The figure also shows that glycine levels are highest in the active season and lower in torpor. This also reflects the human obese state: High BCAA, low glycine.

Desaturase Enzymes Are Increased In Torpor and Heart Disease
The second 2023 paper looked at phospholipid (membrane fats, where a lot of the action is) composition in grizzly bears and dormice during their active seasons and in torpor. I’ll be comparing those numbers to skeletal muscle phospholipids in healthy humans versus those with coronary artery disease (CAD).
Desaturase enzymes leave footprints that we can see in fat composition of different tissues.
For instance, SCD1 converts stearic acid to oleic acid and palmitic acid to palmitoleic acid, creating monounsatured fats (MUFA). When SCD1 activity is high, the ratio of monounsaturated fats to saturated fats increases.
D6D adds a third double bond to linoleic acid (18:2n6). This double bond is the limiting step in converting linoleic acid to arachidonic acid, which can be released from membranes by an enzyme called a phospholipase (PLA), the starting point for oxylipins such as 12-HETE (implicated in heart disease).
I’ve lined up the phospholipid profiles of active vs torpid dormice and healthy humans vs those with CAD using color-matched highlighting so that you can see the pattern:
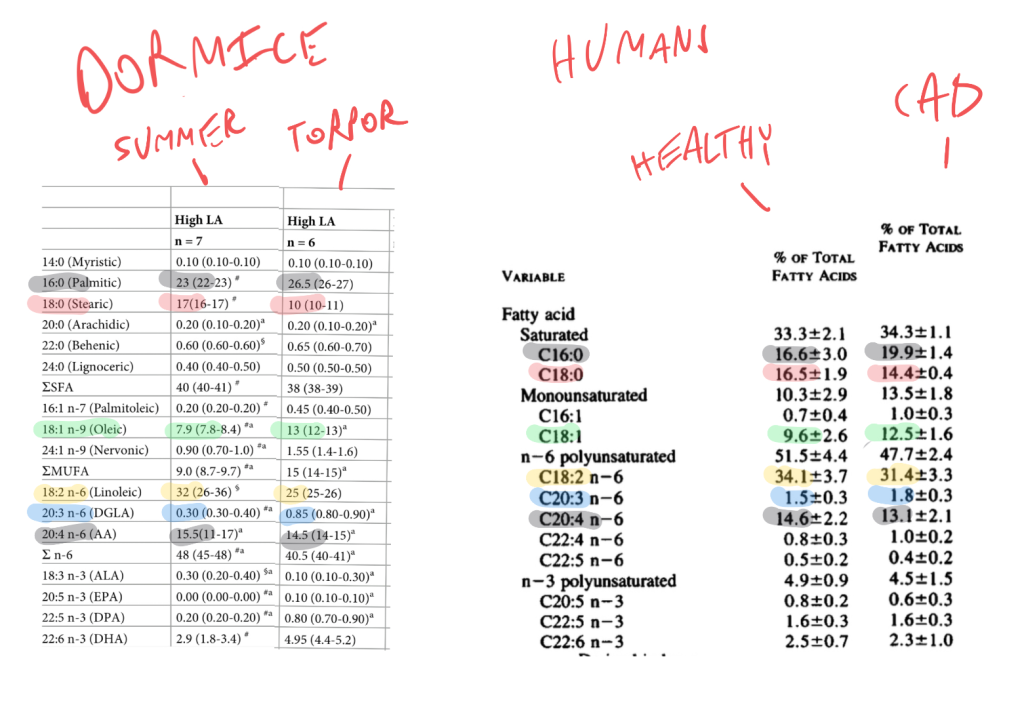
Things that are elevated in both CAD and torpor: palimitic acid, oleic acid (and total MUFA), DGLA.
Things that are lower in torpor/heart disease: stearic acid, linoleic acid and arachidonic acid.
The dormice were fed a high linoleic acid diet in the lab, so we know that seasonal change in linoleic acid and arachidonic acid levels are not due to a dietary shift. Desaturase enzymes are responsible. The researchers calculated the changes in desaturase enzyme activity. SCD16 and SCD18 are indirect measures of SCD1 activity and FADS2 index measures D6D activity.
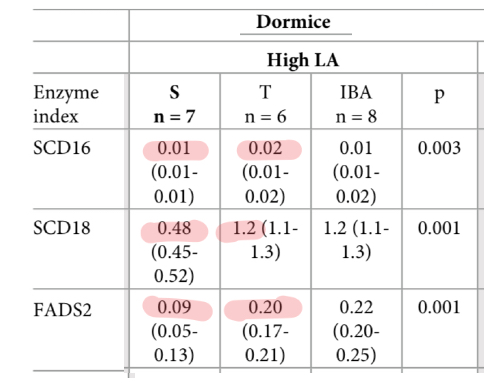
These enzyme activities are directly responsible for declining levels of stearic acid (SCD) and linoleic acid (D6D). SCD1 activity leads to the increase in oleic acid and D6D activity leads to the increase in DGLA, which was highly correlated with insulin resistance in healthy human subjects. Palmitic acid is high because it and oleic acid are the end products of De Novo Lipogenesis.
For more insight on how these enzymes effect phospholipid levels, check out my latest video.
What About The Bears?
The bears look similar, although not quite as straightforward.
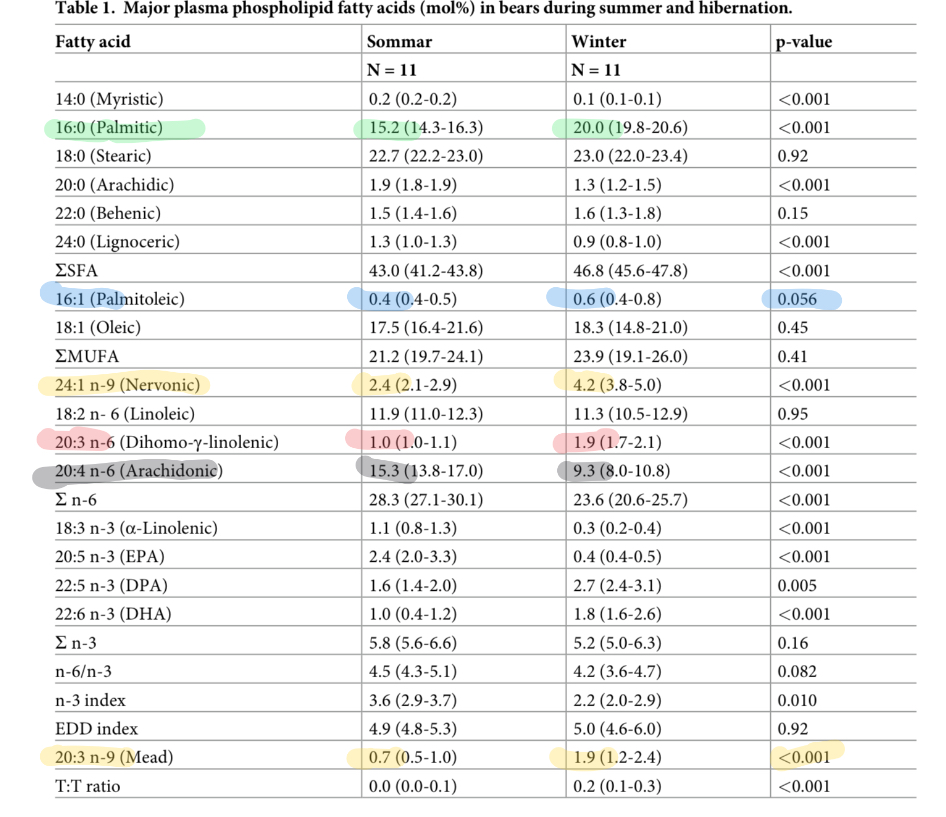
The bears are not so obviously high in MUFA and low in linoleic acid in torpor vs the active season. This may be due to the fact that their relatively larger fat mass has more momentum to buffer shifts between seasons. That is my speculation.
Still, all of the signs of increased desaturation are there. Let’s start with mead acid. This nearly triples between summer and winter. Mead acid is a PUFA that can be made by animals. The starting point is oleic acid, which requires SCD1. The oleic acid is then desaturated by D6D and then D5D. Mead acid requires all three desaturases.
The increases in palmitoleic (16:1) and nervonic acid (24:1) MUFA also attest to increased SCD1 activity. Increased DGLA attests to increased D6D activity.
What Should We Do
I have tried everything from high stearic-acid-relatively-low-carb-diet to very-high-carb-low-fat-high-protein diet and have failed to reverse pre-diabetic levels of morning fasting insulin. All of these diets were low in PUFA and MUFA. In addition to these I have tried several supplements, including but not limited to the SEA and theabrownin I sell on this blog and r-Alpha Lipoic Acid.
All of those approaches worked to some extent, but I still felt like I was fighting against my own metabolism. There are always questions about correlation vs causation, chicken or the egg, what end is the head of the snake. Recent studies have suggested that dietary BCAA are necessary to sustain insulin resistance, so I tried restricting them.u
When I started The Emergence Diet (very high starch, low fat, low BCAA), this all changed. My fasting BG dropped within days and weight started to come off. Controlling BCAA intake has given me a new level of control.
I have repeated many times that BCAA are not ”bad”. Conversely, if you have a torpid metabolism they are likely to be contributing to your insulin resistance. I recommend giving a reduced BCAA diet a try.
Also, if you take one tablet of SEA per day, it may go along way to replacing the stearic acid signalling you’ve lost due to increased SCD1 activity.
Felig, Philip, Errol Marliss, and George F. Cahill. “Plasma Amino Acid Levels and Insulin Secretion in Obesity.” New England Journal of Medicine 281, no. 15 (October 9, 1969): 811–16. https://doi.org/10.1056/NEJM196910092811503.
Heinis, Frazer I., Sophie Alvarez, and Matthew T. Andrews. “Mass Spectrometry of the White Adipose Metabolome in a Hibernating Mammal Reveals Seasonal Changes in Alternate Fuels and Carnitine Derivatives.” Frontiers in Physiology 14 (June 28, 2023): 1214087. https://doi.org/10.3389/fphys.2023.1214087.
Huang, Chin-Chou, Meng-Ting Chang, Hsin-Bang Leu, Wei-Hsian Yin, Wei-Kung Tseng, Yen-Wen Wu, Tsung-Hsien Lin, et al. “Association of Arachidonic Acid-Derived Lipid Mediators with Subsequent Onset of Acute Myocardial Infarction in Patients with Coronary Artery Disease.” Scientific Reports 10, no. 1 (May 15, 2020): 8105. https://doi.org/10.1038/s41598-020-65014-z.
Lackey, Denise E., Christopher J. Lynch, Kristine C. Olson, Rouzbeh Mostaedi, Mohamed Ali, William H. Smith, Fredrik Karpe, et al. “Regulation of Adipose Branched-Chain Amino Acid Catabolism Enzyme Expression and Cross-Adipose Amino Acid Flux in Human Obesity.” American Journal of Physiology-Endocrinology and Metabolism 304, no. 11 (June 1, 2013): E1175–87. https://doi.org/10.1152/ajpendo.00630.2012.
Shao, Chunxuan, Yuting Liu, Hongqiang Ruan, Ying Li, Haifang Wang, Franziska Kohl, Anna V. Goropashnaya, et al. “Shotgun Proteomics Analysis of Hibernating Arctic Ground Squirrels.” Molecular & Cellular Proteomics 9, no. 2 (February 2010): 313–26. https://doi.org/10.1074/mcp.M900260-MCP200.
Strandvik, Birgitta, Abdul Rashid Qureshi, Johanna Painer, Carolina Backman-Johansson, Martin Engvall, Ole Fröbert, Jonas Kindberg, Peter Stenvinkel, and Sylvain Giroud. “Elevated Plasma Phospholipid N-3 Docosapentaenoic Acid Concentrations during Hibernation.” Edited by Manabu Sakakibara. PLOS ONE 18, no. 6 (June 9, 2023): e0285782. https://doi.org/10.1371/journal.pone.0285782.
Yu, Deyang, Nicole E. Richardson, Cara L. Green, Alexandra B. Spicer, Michaela E. Murphy, Victoria Flores, Cholsoon Jang, et al. “The Adverse Metabolic Effects of Branched-Chain Amino Acids Are Mediated by Isoleucine and Valine.” Cell Metabolism 33, no. 5 (May 2021): 905-922.e6. https://doi.org/10.1016/j.cmet.2021.03.025.